SCIENTIFIC RESULTS
Biomimetic magnetic nanoparticles are produced by taking inspiration from nature, i.e., by using magnetosome membrane associated proteins, expressed as recombinant, to in vitro control the nucleation and growth process of magnetic nanoparticles synthesized from aqueous solutions. Special interest have raised MamC-mediated BMNPs, as they have shown in vitro to be effective drug nanocarriers and magnetic hyperthermia agents.

BMNPs SIZE AND MAGNETIC PROPERTIES
The novelty of BMNPs compared to traditional MNPs lies on changes on the size, magnetic properties, and surface properties of the former. These new characteristics of BMNPs are induced by the control over magnetite precipitation exerted by the protein MamC, a magnetosome-associated protein of Magnetococcus marinus MC-1, which is introduced in the reaction mixture from which magnetite forms. MamC chelates Fe cations and acts as template for magnetite nucleation (Ubago et al., 2019), which, in turn, results in superparamagnetic BMNPs nanoparticles, larger (~35-40 nm) than those MNPs chemically produced (<20 nm),(Figure 1).
The size increase allows BMNPs to be superparamagnetic and to display a larger magnetic moment per particle than that displayed by MNPs (Figure 2). This characteristic ensures an enhanced magnetic response once an external magnetic field is applied for guidance and/or concentration, and favors a non-magnetic behavior of BMNPs in the absence of an external magnetic field which prevents BMNPs aggregation (García Rubia et al., 2018).

BMNPs SURFACE PROPERTIES
In terms of surface charge, the advantage of using BMNPs versus MNPs is mainly related to the greater ease in the process of functionalization. MNPs usually have an isoelectric point close to 7, thus they need to be coated to successfully bind the relevant molecule and to keep stable the nano-assembly at physiological pH. Instead, in the case of BMNPs, MamC confers to the nanoparticles surface functional groups, which switch their isoelectric point to ~4.5 (García Rubia et al., 2018; Peigneux et al., 2019); (Figure 3).
This is important, since being the nanoparticle negatively charged at physiological pH, drug binding by electrostatic interaction and nano-assembly stability at this pH value is ensured, while drug release is triggered in acidic environments (as those related to tumors and many bacterial infections), as BMNPs become uncharged, BMNPs behaving as a stimulus-response drug delivery system (Figure 4).

BMNPs AS HYPERTHERMIA AGENTS
Also, these BMNPs have proven to be effective hyperthermia agents under the application of an alternating magnetic field (AMF, Figure 5) or upon irradiation with a laser in the near infrared (NIR), where no collateral tissue damage occurs. This happens regardless whether or not these BMNPs are naked or embedded in liposomes or PLGA that facilitate cell uptake and improve colloidal stability.

BMNPs BIOCOMPATIBILITY
It is equally important to underline the biocompatibility of the BMNPs and the biodistribution studies in mice model, which demonstrate that BMNPs do not accumulate in any organ and they can be magnetically concentrated at the target (Figure 6).

BMNPs APPLICATION IN CANCER
In vitro experiments for antitumoral treatments in different human cell lines (colon, gastric, liver, blood cells and macrophages, among others) show that BMNPs are not toxic in clinical doses. In all these experimental prototypes, the nude BMNPs were cytocompatible and became cytotoxic when they were functionalized with the drug, being the cytotoxic effect similar to obtained at the control experiment with soluble drug.
Moreover, MamC-mediated BMNPs have proven in vivo (mice model) to be suitable nanocarriers for a directed combined therapy (Oltolina et al., 2020). Moreover, they can be embedded in liposomes (Jabalera et al., 2020; Garcia-Pinel et al., 2020) or in PLGA (Vurro et al., 2020) maintaining their functionality and ability for a directed chemotherapy combined with hyperthermia. In fact, recent studies from our group demonstrate that BMNPs, either naked or embedded in liposomes allow the combination of chemotherapy and magnetic hyperthermia in vitro and in vivo in mice models, being that the most effective treatment against tumors compared to free drug and with no secondary effect observed (Figure 7).

BMNPs AS ANTIBACTERIAL AGENTS
We have produced a nanoformulation AS-48_BMNPs, composed of the antimicrobial peptide AS-48 and MamC-mediated biomimetic magnetic nanoparticles, able to mediate a local directed antibacterial therapy combined with local hyperthermia (Figure 8). Our results show that this nanoformulation, either by itself or combined with magnetic hyperthermia, has a strong local bactericide effect on Gram-positive bacteria (Enterococcus faecalis, Enterococcus faecium and Staphylococcus aureus) and, more interestingly, also in Gram-negative bacteria (Pseudomonas aeruginosa, Klebsiella pneumoniae and Escherichia coli). These results represent a step forward in the area offering a nanoassembly that is less prone to generate resistances, that may allow the local treatment of infections and, either by itself or combined with magnetic hyperthermia, offers a potent antibacterial effect against Gram-positive and Gram-negative bacteria.

BMNPs MEDIATE ENZYME RECYCLING
Most of the enzymes used as catalyst to eliminate “bottle necks” in chemical reactions benefit from immobilization, with the goal of preventing their high sensitivity to the extreme conditions, their low stability and their difficulty for recovery and recycling, which influence production costs. Among the typical substrates used for immobilization, the election of magnetic nanoparticles is raising interest due to their high specific surface area and easier separation from the reaction mixture using an external magnetic field, which opens the possibility of enzyme recycling.
Our results show that BMNPs can bind, stabilize enzymes used in biotechnological applications and allow their recycling. Different types of bonding, from electrostatic to covalent can be optimized as a function of the charge and conformation of the relevant enzyme. As an example, AmyKS could be immobilized on BMNPs showing an efficiency of immobilization of 92% of AmyKS. The results show that the immobilization of the enzymes did not extensively alter their functionality and that increased enzyme stability compared to that of the free enzyme. Interestingly, the immobilized amylase was reused for 15 cycles without significant loss of activity upon magnetic recovering of the nanoassemblies (Figure 9). These results suggest the great potential of these nanoassemblies in bio-industry applications.

BMNPs AS ENZYMATIC BIOSENSORS
Sensors to detect trace contaminants in aqueous samples, based on the use of enzymes immobilized on magnetic nanoparticles, have gained interest since they open the possibility of externally controlling, concentrating, recycling and reusing the enzymes, optimizing the cost-efficiency of the process. Our results show that BMNPs can be used to immobilize acetylcholinesterase (AChE) and β-lactamase (BL) to form stable and reusable nanoassemblies capable of detecting trace amounts of organophosphate pesticides (chlorpyrifos) and antibiotics (penicillin G) in aqueous samples.
Our result show that these nanoassemblies were able to detect and quantify trace concentrations of pollutants (1.43 nM for chlorpyrifos, Figure 10, and 0.28 nM for penicillin G). Also, immobilization conferred higher stability to both enzymes, especially in the case of AChE, and allowed the recycling and reusability of BL up to 10 cycles.

BMNPs AS BACTERIAL SENSORS
The development of improved systems for the fast detection of trace amounts of infectious agents is of vital importance. Our results show that BMNPs can be used to concentrate and detect bacteria. As a novelty compared to the existing biosensors based on inorganic magnetic nanoparticles, the surface characteristics of BMNPs allow a direct and efficient electrostatic interaction between microorganisms and nanoparticles without the need of post-production coating of BMNPs. Our results show that BMNPs, without any post-production functionalization, are very efficient binding both Gram positive and Gram negative bacteria and concentrating these microorganisms following upon the application of an external magnetic field. Once concentrated, the target microorganisms (Staphylococcus aureus used here as a model bacterium) can be specifically detected up to bacterial loads as low as 10 CFU/mL by using qPCR (Figure 11). Although the binding is unspecific, the specificity for detection is given by qPCR testing of the attached microorganisms. The system described here, without the need of functionalization, maintains (or improves) the detection limit for S. aureus compared to that obtained by using the Protocol ISO 6888-1:2022 and to that obtained by using antibody-functionalized inorganic magnetic nanoparticles, thus becoming a suitable, cost- and time-effective alternative for bacteria detection in fluid samples.
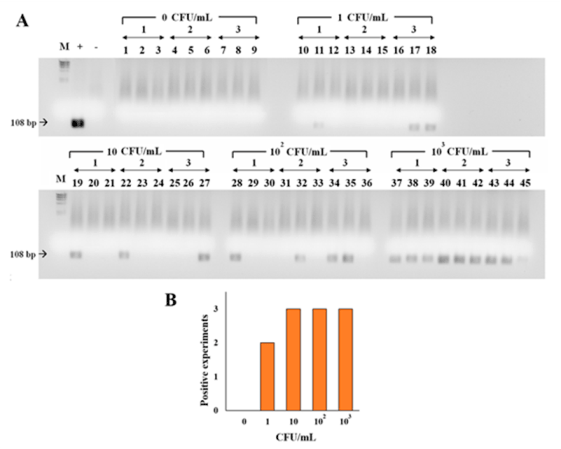
Scientific references supporting results
For updates and more information: https://orcid.org/0000-0002-5645-2079
M. Lázaro, P. Lupiáñez, A. Sola-Leyva, T. Pozo-Gualda, F. Oltolina, M. Jimenez-Carretero, C. Jimenez-Lopez, M.P. Carrasco-Jiménez, G.R. Iglesias, The importance of cell uptake in photothermal treatments mediated by biomimetic magnetic nanoparticles, Colloids and Surfaces B: Biointerfaces, 234, 2024, 113722, https://doi.org/10.1016/j.colsurfb.2023.113722.
Cepero A, Jiménez-Carretero M, Jabalera Y, Gago L, Luque C, Cabeza L, Melguizo C, Jimenez-Lopez C, Prados J. LGR5 as a Therapeutic Target of Antibody-Functionalized Biomimetic Magnetoliposomes for Colon Cancer Therapy. International Journal of Nanomedicine. In Press 2024
Oltolina, F., Santaella Escolano, M.C., Jabalera, Y., Prat, M., Jimenez Lopez, C. (2023) mAb-Functionalized Biomimetic MamC-Mediated-Magnetoliposomes as Drug Delivery Systems for Cancer Therapy. Int. J. Mol. Sci., 24, 13958. https://doi.org/10.3390/
Savchenko, M., Sebastian, V., Lopez-Lopez, M.T., Rodriguez-Navarro, A., Alvarez De Cienfuegos, L., Jimenez-Lopez, C., Gavira, J.A. (2023) Magnetite Mineralization inside Cross-Linked Protein Crystals. Crystal Growth and Design 23 (6), 4032-4040. DOI: 10.1021/acs.cgd.2c01436
Jimenez-Carretero, M., Jabalera, Y., Sola-Leyva, A., Carrasco-Jimenez, M.P., Jimenez-Lopez, C. (2023) Nanoassemblies of acetylcholinesterase and β-lactamase immobilized on magnetic nanoparticles as biosensors to detect pollutants in water. Talanta 258, 124406, . Cited 2 times. DOI: 10.1016/j.talanta.2023.124406
Jimenez-Carretero M., Rodríguez-López J., Ropero-Moreno C., Granada J., Delgado-Martín J., Martinez-Bueno M., Fernandez-Vivas A., Jimenez-Lopez C. (2023) Biomimetic magnetic nanoparticles for bacterial magnetic concentration in liquids and qPCR-detection. Food Control, 147, 109623. https://doi.org/10.1016/j.
Donini, M., Pettinella, F.,Zanella, G., Gaglio, S.C., Laudanna,C., Jimenez-Carretero, M., Jimenez-Lopez, C., Perduca, M., Dusi,S. (2023) Effects of Magnetic Nanoparticles on the Functional Activity of Human Monocytes and Dendritic Cells. J. Mol. Sci. 2023, 24, 1358. https://doi.org/10.3390/
Gaglio SC, Jabalera Y, Montalbán-López M, Millán-Placer AC, Lázaro-Callejón M, Maqueda M, Carrasco-Jimenez MP, Laso A, Aínsa JA, Iglesias GR, Perduca M, López CJ. (2022) Embedding Biomimetic Magnetic Nanoparticles Coupled with Peptide AS-48 into PLGA to Treat Intracellular Pathogens. Pharmaceutics.; 14(12):2744. https://doi.org/10.3390/
Pérez-Huerta A., Cappelli C., Jabalera Y., Prozorov T., Jimenez-Lopez C., Bazylinski D. A. Biogeochemical fingerprinting of magnetotactic bacterial magnetite (2022). Proceedings of the National ACADEMY OF Sciences (PNAS) 119 (31) e2203758119. https://doi.org/10.1073/pnas.
Jabalera, Y., Sola-Leyva. A., Calogero Gaglio, S., Carrasco-Jiménez, M. P., Iglesias, G.R., Perduca, M., Jimenez-Lopez, C. Enhanced Cytotoxic Effect of TAT–PLGA-Embedded DOXO Carried by Biomimetic Magnetic Nanoparticles upon Combination with Magnetic Hyperthermia and Photothermia (2021) Pharmaceutics 13(8), 1168; https://doi.org/10.3390/pharmaceutics13081168
Jabalera, Y., Sola-Leyva, A., Carrasco-Jiménez, M.P., Iglesias, G.R., Jimenez-Lopez, C. Synergistic Photothermal-Chemotherapy based on the use of Biomimetic Magnetic Nanoparticles. Pharmaceutics (2021), 13, 625. https://doi.org/10.3390/pharmaceutics13050625.
Salem, K., Jabalera, Y., Puentes-Pardo, J.D., Vilchez-Garcia, J., Sayari, A., Hmida-Sayari, A., Jimenez-Lopez, C.,* Perduca, M. Enzyme Storage and Recycling: Nanoassemblies of α-Amylase and Xylanase Immobilized on Biomimetic Magnetic Nanoparticles (2021) ACS Sustainable Chemistry & Engineering. DOI: 10.1021/acssuschemeng.0c08300.
Vurro, F., Jabalera, Y., Mannucci, S., Glorani, G., Sola-Leyva, A., Gerosa, M., Romeo, A., Romanelli, M.G., Malatesta, M., Calderan, L., Iglesias, G.R., Carrasco-Jiménez, M.P., Jimenez-Lopez, C., Perduca, M. (2021) Improving the Cellular Uptake of Biomimetic Magnetic Nanoparticles. Nanomaterials, 11, 766. DOI: 10.3390/nano11030766.
Sola-Leyva, A., Jabalera, Y., Chico-Lozano, M.A., Carrasco-Jiménez, M.P., Iglesias, G.R., Jimenez-Lopez, C. Reactive oxygen species (ROS) production in HepG2 cancer cell line through the application of localized alternating magnetic field (2020) Journal of Materials Chemistry B, 8 (34), pp. 7667-7676. DOI: 10.1039/d0tb01306d.
Oltolina, F., Peigneux, A., Colangelo, D., Clemente, N., D’urso, A., Valente, G., Iglesias, G.R., Jiménez-Lopez, C., Prat, M. Biomimetic magnetite nanoparticles as targeted drug nanocarriers and mediators of hyperthermia in an experimental cancer model (2020) Cancers, 12 (9), art. no. 2564, pp. 1-25. DOI: 10.3390/cancers12092564.
Jabalera, Y., Oltolina, F., Peigneux, A., Sola-Leyva, A., Carrasco-Jiménez, M.P., Prat, M., Jimenez-Lopez, C., Iglesias, G.R. Nanoformulation design including MamC-mediated biomimetic nanoparticles allows the simultaneous application of targeted drug delivery and magnetic hyperthermia (2020) Polymers, 12 (8), art. no. 1832. DOI: 10.3390/POLYM12081832.
Peigneux, A., Glitscher, E.A., Charbaji, R., Weise, C., Wedepohl, S., Calderón, M., Jimenez-Lopez, C., Hedtrich, S. Protein corona formation and its influence on biomimetic magnetite nanoparticles (2020) Journal of Materials Chemistry B, 8 (22), pp. 4870-4882. DOI: 10.1039/c9tb02480h.
Garcia-Pinel, B., Jabalera, Y., Ortiz, R., Cabeza, L., Jimenez-Lopez, C., Melguizo, C., Prados, J. Biomimetic magnetoliposomes as oxaliplatin nanocarriers: In vitro study for potential application in colon cancer (2020) Pharmaceutics, 12 (6), art. no. 589, pp. 1-20. DOI: 10.3390/pharmaceutics12060589.
Peigneux, A., Puentes-Pardo, J.D., Rodríguez-Navarro, A.B., Hincke, M.T., Jimenez-Lopez, C. Development and characterization of magnetic eggshell membranes for lead removal from wastewater (2020) Ecotoxicology and Environmental Safety, 192, art. no. 110307. DOI: 10.1016/j.ecoenv.2020.110307.
Jabalera, Y., Oltolina, F., Prat, M., Jimenez-Lopez, C., Fernández-Sánchez, J.F., Choquesillo-Lazarte, D., Gómez-Morales, J. Eu-doped citrate-coated carbonated apatite luminescent nanoprobes for drug delivery (2020) Nanomaterials, 10 (2), art. no. 199. DOI: 10.3390/nano10020199.
Jabalera, Y., Fernández-Vivas, A., Iglesias, G.R., Delgado, Á.V., Jimenez-Lopez, C. Magnetoliposomes of mixed biomimetic and inorganic magnetic nanoparticles as enhanced hyperthermia agents (2019) Colloids and Surfaces B: Biointerfaces, 183, art. no. 110435. DOI: 10.1016/j.colsurfb.2019.110435.
Jabalera, Y., Garcia-Pinel, B., Ortiz, R., Iglesias, G., Cabeza, L., Prados, J., Jimenez-Lopez, C., Melguizo, C. Oxaliplatin–biomimetic magnetic nanoparticle assemblies for colon cancer-targeted chemotherapy: An in vitro study (2019) Pharmaceutics, 11 (8), art. no. 395. DOI: 10.3390/pharmaceutics11080395.
Jabalera, Y., Sola-Leyva, A., Peigneux, A., Vurro, F., Iglesias, G.R., Vilchez-Garcia, J., Pérez-Prieto, I., Aguilar-Troyano, F.J., López-Cara, L.C., Carrasco-Jiménez, M.P., Jimenez-Lopez, C. Biomimetic magnetic nanocarriers drive choline kinase alpha inhibitor inside cancer cells for combined chemo-hyperthermia therapy (2019) Pharmaceutics, 11 (8), art. no. 408. DOI: 10.3390/pharmaceutics11080408.
Peigneux, A., Oltolina, F., Colangelo, D., Iglesias, G.R., Delgado, A.V., Prat, M., Jimenez-Lopez, C. Functionalized Biomimetic Magnetic Nanoparticles as Effective Nanocarriers for Targeted Chemotherapy (2019) Particle and Particle Systems Characterization, 36 (6), art. no. 1900057. DOI: 10.1002/ppsc.201900057.
Ubago-Rodríguez, A., Casares Atienza, S., Fernández-Vivas, A., Peigneux, A., Jabalera, Y., De La Cuesta-Rivero, M., Jimenez-Lopez, C., Azuaga Fortes, A.I. Structure-Function of MamC Loop and Its Effect on the in Vitro Precipitation of Biomimetic Magnetite Nanoparticles (2019) Crystal Growth and Design, 19 (5), pp. 2927-2935. DOI: 10.1021/acs.cgd.9b00150.
Iglesias, G.R., Jabalera, Y., Peigneux, A., Fernández, B.L.C., Delgado, Á.V., Jimenez-Lopez, C. Enhancement of magnetic hyperthermia by mixing synthetic inorganic and biomimetic magnetic nanoparticles (2019) Pharmaceutics, 11 (6), art. no. 273. DOI: 10.3390/pharmaceutics11060273.
García Rubia, G., Peigneux, A., Jabalera, Y., Puerma, J., Oltolina, F., Elert, K., Colangelo, D., Gómez Morales, J., Prat, M., Jimenez-Lopez, C. PH-Dependent Adsorption Release of Doxorubicin on MamC-Biomimetic Magnetite Nanoparticles (2018) Langmuir, 34 (45), pp. 13713-13724. DOI: 10.1021/acs.langmuir.8b03109.